[ad_1]
As humans warm the planet, biodiversity is plummeting. These two global crises are connected in multiple ways. But the details of the intricate feedback loops between biodiversity decline and climate change are astonishingly under-studied.
It is well known that climate extremes such as droughts and heatwaves can have devastating impacts on ecosystems and, in turn, that degraded ecosystems have a reduced capacity to protect humanity against the social and physical impacts of such events. Yet only a few such relationships have been probed in detail. Even less well known is whether biodiversity-depleted ecosystems will also have a negative effect on climate, provoking or exacerbating weather extremes.
For us, a group of researchers living and working mainly in Central Europe, the wake-up call was the sequence of heatwaves of 2018, 2019 and 2022. It felt unreal to watch a floodplain forest suffer drought stress in Leipzig, Germany. Across Germany, more than 380,000 hectares of trees have now been damaged (see go.nature.com/3etrrnp; in German), and the forestry sector is struggling with how to plan restoration activities over the coming decades1. What could have protected these ecosystems against such extremes? And how will the resultant damage further impact our climate?
Nature-based solutions can help cool the planet — if we act now
In June 2021, the Intergovernmental Panel on Climate Change (IPCC) and the Intergovernmental Science-Policy Platform on Biodiversity and Ecosystem Services (IPBES) published their first joint report2, acknowledging the need for more collaborative work between these two domains. And some good policy moves are afoot: the new EU Forest Strategy for 2030, released in July 2021, and other high-level policy initiatives by the European Commission, formally recognize the multifunctional value of forests, including their role in regulating atmospheric processes and climate. But much more remains to be done.
To thoroughly quantify the risk that lies ahead, ecologists, climate scientists, remote-sensing experts, modellers and data scientists need to work together. The upcoming meeting of the United Nations Convention on Biological Diversity in Montreal, Canada, in December is a good opportunity to catalyse such collaboration.
Buffers and responses
When lamenting the decline in biodiversity, most people think first about the tragedy of species driven to extinction. There are more subtle changes under way, too.
For instance, a study across Germany showed that over the past century, most plant species have declined in cover, with only a few increasing in abundance3. Also affected is species functionality4 — genetic diversity, and the diversity of form and structure that can make communities more or less efficient at taking up nutrients, resisting heat or surviving pathogen attacks.
When entire ecosystems are transformed, their functionality is often degraded. They are left with less capacity to absorb pollution, store carbon dioxide, soak up water, regulate temperature and support vital functions for other organisms, including humans5. Conversely, higher levels of functional biodiversity increase the odds of an ecosystem coping with unexpected events, including climate extremes. This is known as the insurance effect6.
The effect is well documented in field experiments and modelling studies. And there is mounting evidence of it in ecosystem responses to natural events. A global synthesis of various drought conditions showed, for instance, that forests were more resilient when trees with a greater diversity of strategies for using and transporting water lived together7.
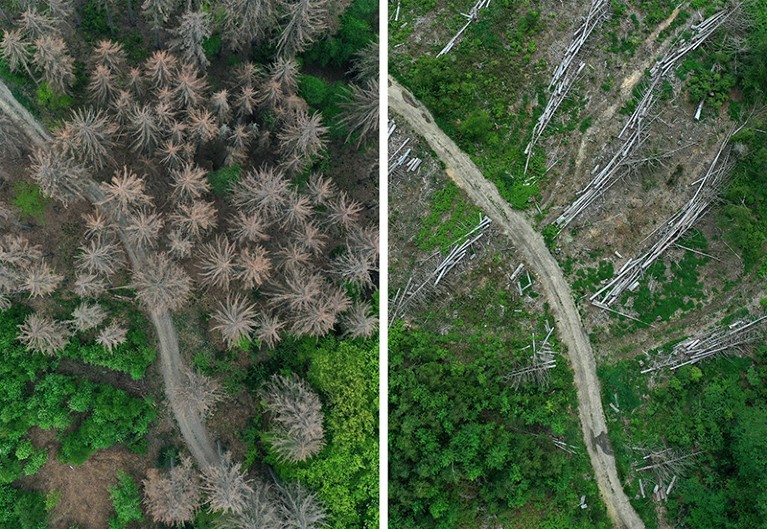
Dead trees near Iserlohn, Germany, in April 2020 (left) and after felling in June 2021 (right).Credit: Ina Fassbender/AFP via Getty
However, biodiversity cannot protect all ecosystems against all kinds of impacts. In a study this year across plots in the United States and Canada, for example, mortality was shown to be higher in diverse forest ecosystems8. The proposed explanation for this unexpected result was that greater biodiversity could also foster more competition for resources. When extreme events induce stress, resources can become scarce in areas with high biomass and competition can suddenly drive mortality, overwhelming the benefits of cohabitation. Whether or not higher biodiversity protects an ecosystem from an extreme is highly site-specific.
Some plants respond to drought by reducing photosynthesis and transpiration immediately; others can maintain business as usual for much longer, stabilizing the response of the ecosystem as a whole. So the exact response of ecosystems to extremes depends on interactions between the type of event, plant strategies, vegetation composition and structure.
Which plant strategies will prevail is hard to predict and highly dependent on the duration and severity of the climatic extreme, and on previous extremes9. Researchers cannot fully explain why some forests, tree species or individual plants survive in certain regions hit by extreme climate conditions, whereas entire stands disappear elsewhere10. One study of beech trees in Germany showed that survival chances had a genomic basis11, yet it is not clear whether the genetic variability present in forests will be sufficient to cope with future conditions.
And it can take years for ecosystem impacts to play out. The effects of the two consecutive hot drought years, 2018 and 2019, were an eye-opener for many of us. In Leipzig, tree growth declined, pathogens proliferated and ash and maple trees died. The double blow, interrupted by a mild winter, on top of the long-term loss of soil moisture, led to trees dying at 4–20 times the usual rate throughout Germany, depending on the species (see go.nature.com/3etrrnp; in German). The devastation peaked in 2020.
Ecosystem changes can also affect atmospheric conditions and climate. Notably, land-use change can alter the brightness (albedo) of the planet’s surface and its capacity for heat exchange. But there are more-complex mechanisms of influence.
Vegetation can be a source or sink for atmospheric substances. A study published in 2020 showed that vegetation under stress is less capable of removing ozone than are unstressed plants, leading to higher levels of air pollution12. Pollen and other biogenic particles emitted from certain plants can induce the freezing of supercooled cloud droplets, allowing ice in clouds to form at much warmer temperatures13, with consequences for rainfall14. Changes to species composition and stress can alter the dynamics of these particle emissions. Plant stress also modifies the emission of biogenic volatile organic gases, which can form secondary particles. Wildfires — enhanced by drought and monocultures — affect clouds, weather and climate through the emission of greenhouse gases and smoke particles. Satellite data show that afforestation can boost the formation of low-level, cooling cloud cover15 by enhancing the supply of water to the atmosphere.
Research priorities
An important question is whether there is a feedback loop: will more intense, and more frequent, extremes accelerate the degradation and homogenization of ecosystems, which then, in turn, promote further climate extremes? So far, we don’t know.
One reason for this lack of knowledge is that research has so far been selective: most studies have focused on the impacts of droughts and heatwaves on ecosystems. Relatively little is known about the impacts of other kinds of extremes, such as a ‘false spring’ caused by an early-season bout of warm weather, a late spring frost, heavy rainfall events, ozone maxima, or exposure to high levels of solar radiation during dry, cloudless weather.
Researchers have no overview, much less a global catalogue, of how each dimension of biodiversity interacts with the full breadth of climate extremes in different combinations and at multiple scales. In an ideal world, scientists would know, for example, how the variation in canopy density, vegetation age, and species diversity protects against storm damage; and whether and how the diversity of canopy structures controls atmospheric processes such as cloud formation in the wake of extremes. Researchers need to link spatiotemporal patterns of biodiversity with the responses of ecosystem processes to climate extremes.
Biodiversity needs every tool in the box: use OECMs
Creating such a catalogue is a huge challenge, particularly given the more frequent occurrence of extremes with little or no precedent16. Scientists will also need to account for the increasing likelihood of pile-ups of climate stressors. The ways in which ecosystems respond to compound events17 could be quite different. Researchers will have to study which facets of biodiversity (genetic, physiological, structural) are required to stabilize ecosystems and their functions against these onslaughts.
There is at least one piece of good news: tools for data collection and analysis are improving fast, with huge advances over the past decade in satellite-based observations for both climate and biodiversity monitoring. The European Copernicus Earth-observation programme, for example — which includes the Sentinel 1 and 2 satellite fleet, and other recently launched missions that cover the most important wavelengths of the electromagnetic spectrum — offer metre-scale resolution observations of the biochemical status of plants and canopy structure. Atmospheric states are recorded in unprecedented detail, vertically and in time.
Scientists must now make these data interoperable and integrate them with in situ observations. The latter is challenging. On the ground, a new generation of data are being collected by researchers and by citizen scientists18. For example, unique insights into plant responses to stress are coming from time-lapse photography of leaf orientation; accelerometer measures of movement patterns of stems have been shown to provide proxies for the drought stress of trees19.
High-quality models are needed to turn these data into predictions. The development of functional ‘digital twins’ of the climate system is now in reach. These models replicate hydrometeorological processes at the metre scale, and are fast enough to allow for rapid scenario development and testing20. The analogous models for ecosystems are still in a more conceptual phase. Artificial-intelligence methods will be key here, to study links between climate extremes and biodiversity.
Researchers can no longer afford to track global transformations of the Earth system in disciplinary silos. Instead, ecologists and climate scientists need to establish a joint agenda, so that humanity is properly forewarned: of the risks of removing biodiversity buffers against climate extremes, and of the risk of thereby amplifying these extremes.
[ad_2]